Distinct and opposite diversifying activities of terminal transferase splice variants
The short splice variant of mouse terminal deoxynucleotidyl transferase (TdTS) catalyzes the addition of nontemplated nucleotides (N addition) at the coding joins of B cell and T cell antigen receptor genes. However, the activity and function of the long isoform of TdT (TdTL) have not been determined. We show here, in vitro and in vivo, that TdTL is a 3′→5′ exonuclease that catalyzes the deletion of nucleotides at coding joins. These findings suggest that the two TdT isoforms may act in concert to preserve the integrity of the variable region of antigen receptors while generating diversity.
This is a preview of subscription content, access via your institution
Access options
Subscribe to this journal
Receive 12 print issues and online access
206,07 € per year
only 17,17 € per issue
Buy this article
- Purchase on SpringerLink
- Instant access to full article PDF
Prices may be subject to local taxes which are calculated during checkout
Similar content being viewed by others
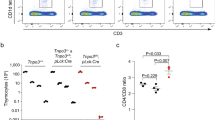
Tnpo3 controls splicing of the pre-mRNA encoding the canonical TCR α chain of iNKT cells
Article Open access 20 June 2023
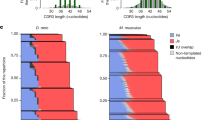
Origin and evolutionary malleability of T cell receptor α diversity
Article Open access 21 June 2023
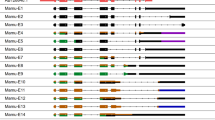
Alternative splicing and genetic variation of mhc-e: implications for rhesus cytomegalovirus-based vaccines
Article Open access 19 December 2022
Accession codes
Accessions
GenBank/EMBL/DDBJ
References
- Hozumi, N. & Tonegawa, S. Evidence for somatic rearrangement of immunoglobulin genes coding for variable and constant regions. Proc. Natl. Acad. Sci. USA73, 3628–3632 (1976). ArticleCASGoogle Scholar
- Lewis, M. S. The mechanism of V(D)J joining: Lessons from molecular, immunological, and comparative analyses. Adv. Immunol.56, 27–150 (1994). ArticleCASGoogle Scholar
- Fugmann, S. D. et al. The RAG proteins and V(D)J recombination: complexes, ends, and transposition. Ann. Rev. Immunol.18, 497–527 (2000). ArticleGoogle Scholar
- Oettinger, M. A. V(D)J recombination: on the cutting edge. Curr. Opin. Cell Biol.11, 325–329 (1999). ArticleCASGoogle Scholar
- Arstilla, P. T. et al. A direct estimate of the human αβ T cell receptor diversity. Science286, 958–961 (1999). ArticleGoogle Scholar
- Cabaniols, J. P. et al. Most αβ T cell receptor diversity is due to terminal deoxynucleotidyl transferase. J. Exp. Med.194, 1385–1390 (2001). ArticleCASGoogle Scholar
- Komori, T., Okada, A., Stewart, V. & Alt. F. W. Lack of N regions in antigen receptor variable region genes of TdT-deficient lymphocytes. Science261, 1171–1175 (1993). ArticleCASGoogle Scholar
- Gilfillan, S., Dierich, A., Lemeur, M., Benoist, C. & Mathis, D. Mice lacking TdT: mature animals with an immature lymphocyte repertoire. Science261, 1175–1178 (1993). ArticleCASGoogle Scholar
- Doyen, N. et al. Differential splicing in mouse thymus generates two forms of terminal deoxynucleotidyl transferase. Nucleic Acids Res.21, 1187–1191 (1993). ArticleCASGoogle Scholar
- Koiwai, O. et al. Isolation and characterization of bovine and mouse terminal deoxynucleotidyl transferase cDNAs expressible in mammalian cells. Nucleic Acids Res.14, 5777–5792 (1986). ArticleCASGoogle Scholar
- Farrar, Y. J., Evans, R. B., Beach, C. M. & Coleman, M. S. Interactions of photoactive DNAs with terminal deoxynucleotidyl transferase: identification of peptides in the DNA binding domain. Biochemistry30, 3075–3082 (1991). ArticleCASGoogle Scholar
- Yang, B., Gathy, K. N. & Coleman, M. S. Mutational analysis of residues in the nucleotide binding domain of human terminal deoxynucleotidyl transferase. J. Biol. Chem.269, 11859–11868 (1994). CASPubMedGoogle Scholar
- Benedict, C. L. & Kearney, J. F. Increased junctional diversity in fetal B cells results in a loss of protective anti-phosphorylcholine antibodies in adult mice. Immunity5, 607–617 (1999). ArticleGoogle Scholar
- Bentolila, L. A. et al. The two isoforms of mouse terminal deoxynucleotidyl transferase differ in both the ability to add N regions and subcellular localization. EMBO J.14, 4221–4229 (1995). ArticleCASGoogle Scholar
- Feeney, A. J. Lack of N regions in fetal and neonatal mouse immunoglobulin V-D-J junctional sequences. J. Exp. Med.172, 1377–1390 (1990). ArticleCASGoogle Scholar
- Lafaille, J., DeCloux, A., Bonneville, M, Takagaki, Y. & Tonegawa, S. Junctional sequences of T cell receptor γδ genes: implications for γδ T cell lineages and for a novel intermediate of V-(D)-J joining. Cell59, 859–870 (1989). ArticleCASGoogle Scholar
- McVay, L. D., Carding, S. R., Bottomly, K. & Hayday, A. C. Regulated expression and structure of T cell receptor γ/δ transcripts in human thymic ontogeny. EMBO J.10, 83–91 (1991). ArticleCASGoogle Scholar
- Schwager, J., Burckert, N., Courtet, M. & Du Pasquier, L. The ontogeny of diversification at the immunoglobulin heavy chain locus in Xenopus. EMBO J.10, 2461–2470 (1991). ArticleCASGoogle Scholar
- Carlsson, L., Overmo, C. & Holmberg, D. Developmentally controlled selection of antibody genes: characterization of individual VH7183 genes and evidence for stage-specific somatic diversification. Eur. J. Immunol.22, 71–78 (1992). ArticleCASGoogle Scholar
- Medina, C. A. & Teale, J. M. Restricted κ chain expression in early ontogeny: biased utilization of Vκ exons and preferential Vκ-Jκ recombinations. J. Exp. Med.177, 1317–1330 (1993). ArticleCASGoogle Scholar
- Rothenberg, E. & Triglia, D. Clonal proliferation unlinked to terminal deoxynucleotidyl transferase synthesis in thymocytes of young mice. J. Immunol.130, 1627–1633 (1983). CASPubMedGoogle Scholar
- Benedict, C. L., Gilfillan, S. & Kearney, J. F. The long isoform of terminal deoxynucleotidyl transferase enters the nucleus and, rather than catalyzing nontemplated nucleotide addition, modulates the catalytic activity of the short isoform. J. Exp. Med.193, 89–99 (2001). ArticleCASGoogle Scholar
- Melchers, F. et al. Positive and negative selection events during B lymphopoiesis. Curr. Opin. Immunol.7, 214–227 (1995). ArticleCASGoogle Scholar
- Grawunder, U. et al. Down-regulation of RAG1 and RAG2 gene expression in pre-B cells after functional immunoglobulin heavy chain rearrangement. Immunity3, 601–608 (1995). ArticleCASGoogle Scholar
- Mazur, D. J. & Perrino, F. W. Identification and expression of the TREX1 and TREX2 cDNA sequences encoding mammalian 3′→5′ exonucleases. J. Biol. Chem.274, 19655–19660 (1999). ArticleCASGoogle Scholar
- Schlissel, M. S. Structure of nonhairpin coding-end DNA breaks in cells undergoing V(D)J recombination. Mol. Cell Biol.18, 2029–2037 (1998). ArticleCASGoogle Scholar
- Livák, F. L. & Schatz, D. G. Identification of V(D)J recombination coding end intermediates in normal thymocytes. J. Mol. Biol.267, 1–9 (1997). ArticleGoogle Scholar
- Roth, D. B., Zhu, C. & Gellert, M. Characterization of broken DNA molecules associated with V(D)J recombination. Proc. Natl. Acad. Sci. USA90, 10788–10972 (1993). ArticleCASGoogle Scholar
- Schlissel, M. S., Constantinescu, A., Morrows, T., Baxter, M. & Peng, A. Double-strand signal sequence breaks in V(D)J recombination are blunt, 5′-phosphorylated, RAG-dependent, and cell-cycle regulated. Genes Dev.7, 2520–2532 (1993). ArticleCASGoogle Scholar
- Hoss, M. et al. A human DNA editing enzyme homologous to the Escherichia coli Dna/Q/MutD protein. EMBO J.18, 3868–3875 (1999). ArticleCASGoogle Scholar
- Bernad, A., Blanco, L., Lazaro, J. M., Martin, G. & Salas, M. A conserved 3′→5′ exonuclease active site in prokaryotic and eukaryotic DNA polymerases. Cell59, 219–228 (1989). ArticleCASGoogle Scholar
- Reha-Krantz, L. J. et al. DNA polymerization in the absence of exonucleolytic proofreading: In vivo and in vitro studies. Proc. Natl. Acad. Sci. USA88, 2417–2421 (1991). ArticleCASGoogle Scholar
- Derbyshire, V., Grindley, N. D. & Joyce, C. M. The 3′→5′ exonuclease of DNA polymerase I of Escherichia coli: contribution of each amino acid at the active site to the reaction. EMBO J.1, 17–24 (1991). ArticleGoogle Scholar
- Reha-Krantz, L. J. & Nonay, R. L. Genetic and biochemical studies of bactriophage T4 DNA polymerase 3′→5′-exonuclease activity. J. Biol. Chem.268, 27100–27108 (1983). Google Scholar
- Wang, J., Yu, P., Lin, T. C., Konigsberg, W. H. & Steitz, T. A. Crystal structures of an NH2-terminal fragment of T4 DNA polymerase and its complexes with single-stranded DNA and with divalent metal ions. Biochemistry35, 8110–8119 (1996). ArticleCASGoogle Scholar
- Purugganan, M. M., Shah, S., Kearney, J. F. & Roth, D. B. Ku80 is required for addition of N nucleotides to V(D)J recombination junctions by terminal deoxynucleotidyl transferase. Nucleic Acids Res.29, 1638–1646 (2001). ArticleCASGoogle Scholar
- Takahara, K. et al. Alternative splicing of bovine terminal deoxynucleotidyl transferase cDNA. Biosci. Biotech. Biochem.58, 786–787 (1994). ArticleCASGoogle Scholar
- Boulé, J. B., Rougeon, F. & Panicolaou, C. Comparison of the two murine deoxynucleotidyl transferase. J. Biol. Chem.275, 28984–28988 (2000). ArticleGoogle Scholar
- Fang, W. et al. Frequent aberrant immunoglobulin gene rearrangements in pro-B cells revealed by a bcl-xL transgene. Immunity4, 291–299 (1996). ArticleCASGoogle Scholar
- Victor, K. D., Vu, K. & Feeney, A. J. Limited junctional diversity in κ light chains. J. Immnunol.152, 3467 (1994).
- Whitcomb, E. A. & Brodeur, P. H. Rearrangement and selection in the developing Vκ repertoire of the mouse: an analysis of the usage of two Vκ gene. J. Immunol.160, 4904–4913 (1998). CASPubMedGoogle Scholar
- Levine, M. TdT effects during κ light chain recombination and influence on BCR selection. Thesis, Yale Univ. (2000).
Acknowledgements
We thank S. Gilfillan and D. Mathis for the TdTL and TdTS cDNA clones; D. J. Mazure and F. W. Perrino for hTREX2; M. Oettinger for full-length RAG-1 and RAG-2; L. Gartland for FACS; X. Y. Liu for technical assistance; M. A. Anderson, D. S. Nelson, P. D. Burrows for helpful discussions; and A. Brookshire for preparing this manuscript. Supported by NIH grants AI 523133, AI 07051, AI36420 and the Howard Hughes Medical Institute (D. B. R.).
Author information
- Mary M. Purugganan Present address: Cain Project in Engineering and Professional Communication, Rice University, P.O. Box 1892, MS345, Houston, TX, 77251-1892, USA
Authors and Affiliations
- Division of Developmental and Clinical Immunology, Department of Microbiology, The University of Alabama at Birmingham, 378 Wallace Tumor Institute, Birmingham, 35294, AL, USA To-Ha Thai & John F. Kearney
- Department of Immunology, M929, Baylor College of Medicine, 1 Baylor Plaza, Houston, 77030, TX, USA Mary M. Purugganan & David B. Roth
- To-Ha Thai